Purpose
In the field of rectal cancer, the risk of loco-regional recurrence has been reduced by the introduction of total mesorectal excision (TME) surgery that has significantly improved loco-regional control [1-4]. Neoadjuvant (chemo) radiotherapy has further decreased the chance of loco-regional recurrence in patients with locally advanced rectal cancer (LARC) [5-8]. Nevertheless, recurrences still occur and prove difficult to treat. In both primary and locally recurrent rectal cancer (LRRC), a microscopically radical (R0) resection margin has shown to be extremely important in improving local control [4, 7, 9-11].
Intra-operative radiotherapy (IORT) can further improve local control in patients with locally advanced or recurrent rectal cancer (LARRC), by delivering a high-dose of radiation to a limited at-risk volume, thus reducing excessive toxicity. By applying IORT to the at-risk resection margin, the remaining microscopic disease may be treated intra-operatively [12, 13]. Intra-operative radiotherapy is mainly performed with electrons (IOERT), high-dose-rate (HDR) brachytherapy (IOBT), or low-kV X-rays (orthovoltage).
A recent article compared the results of IOERT vs. IOBT for microscopically irradical (R1) resections in patients with LARRC [14]. An improvement in local recurrence-free survival (LRFS) was observed, favoring IOBT. Not only does this suggest that IORT affects LRFS in general, but the difference in favor of IOBT raises the question of why this might be so. Although many factors may have contributed to this fact, such as different patient populations, larger irradiated volumes, different operating times, and various applicator types, one striking difference is a higher surface dose of IOBT compared with IOERT [15]. Since the surgical resection surface is the site where potential microscopic disease remains, it has been suggested that different surface doses may have contributed strongly to the results. In a second article, the two techniques were compared, and the IOERT technique was adapted to increase the surface dose to approximate that of IOBT (Figure 1) [16]. First, a bolus of tissue equivalent polymethyl methacrylate (PMMA) was added to the IOERT applicator exit to move maximum dose to the tissue surface (Figure 2). The dose was then re-scaled to increase the surface dose to approximate that of IOBT. The prescribed dose (10 Gy) of adjusted dose curve was still at 9 mm, as in the original dose curve of IOERT. In this way, the dosimetric difference between IOERT and IOBT was reduced at the surface. One point of concern, however, is the potential additional toxicity caused by the higher surface dose. As described in a study by Verrijssen et al., this was not expected, as the published literature on IOBT, which is characterized by a higher surface dose, does not show an increase in toxicity [16]. The present work aimed to confirm this hypothesis.
Fig. 1
Graph showing current situation for IOERT and IOBT in absolute dose at depth for IOBT (solid line) and IOERT (long dashed line). Short dashed line illustrates the IOERT curve after applying a bolus, but before re-scaling. Dotted line shows the IOERT curve with a bolus and re-scaling to deliver 10 Gy at 9 mm
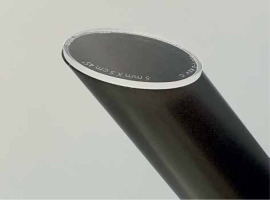
Material and methods
Patient selection
All patients who underwent surgery in combination with IOERT for LARRC at Catharina Hospital Eindhoven (CHE) from September 1, 2019 to July 31, 2023, were selected. Patients treated until August 31, 2021 were included in the control cohort. Patients treated from September 1, 2021 onwards were included in the intervention group, after the adapted technique was introduced. Patients with a re-recurrence were excluded. Data of LRRC patients were extracted from a prospectively maintained database, and were updated from electronic patient records if necessary. Data of LARC patients were retrieved from medical records. Information recorded included baseline characteristics, neoadjuvant treatment, surgery, outcome, and follow-up. Follow-up was completed until August 29, 2023. The study was approved by the local medical ethics committee (approval No.: nWMO-2022.130).
Neoadjuvant treatment
All patients received neoadjuvant (chemo) radiotherapy. For LARC, treatment regimens consisted of either short course radiotherapy (5 × 5 Gy) or full course chemoradiotherapy (CRT) (25 × 2 Gy), with concomitant capecitabine. Locally recurrent rectal cancer patients were given either full course CRT or chemo re-irradiation (15 × 2 Gy), with concomitant capecitabine. Selected LARRC patients received induction chemotherapy prior to (chemo) radiotherapy, which generally consisted of either 3-4 courses of CAPOX (capecitabine and oxaliplatin) or 4-6 courses of FOLFOX (5-FU, leucovorin, and oxaliplatin).
Surgery was categorized as follows: abdomino-perineal resection (APR), low-anterior resection (LAR), total exenteration (TE, including a resection of the rectum, bladder, prostate, and vesicles in male patients, or ovaries, vagina, and uterus in female patients), and a tumor resection not otherwise specified (n.o.s.), i.e., tumor resection without formal bowel resection. All resections performed in addition to a formal bowel resection were documented separately (i.e., in the case of a TE, resections of the bladder, prostate, and vesicles were also noted separately).
Intra-operative electron radiotherapy
A preliminary assessment of IOERT indication is performed for all patients undergoing either curative surgery or surgery in a palliative setting, for maximal local control of LARRC. This is done during an expert multidisciplinary tumor board, including at least an oncologic surgeon, medical oncologist, radiation oncologist, and radiologist, where staging at baseline and after neoadjuvant treatment is discussed. Perioperatively, the IOERT indication is re-established by a surgeon and radiation oncologist. In both instances, a clinical suspicion of either narrow or involved resection margins is considered an IOERT indication. Additional frozen sections of excised tissue can be used perioperatively to aid decision-making, but are not required. The IOERT dose and irradiation site are also determined perioperatively, targeting the area at-risk for R1 resection. IOERT is administered using Mobetron 2000 linear accelerator (IntraOp Medical, Sunnyvale California, USA), with energy of either 6, 9, or 12 MeV. Applicators with a diameter of five to ten centimeters can be used, with bevel angles of 0, 30, or 45 degrees. The dose-rate is set at around 10 Gy/min. The IOERT dose previously depended on the depth of tissue considered at-risk for R1 resection, and was prescribed (at depth) at 10 Gy, 12.5 Gy, or 14.4 Gy to 90% isodose surface. From September 1, 2021 onwards, all patients received a dose of 10 Gy at the target depth, which corresponded to a dose between 15.5 Gy and 16.5 Gy at the tissue surface (Figure 1) [16]. The following IOERT variables were reported for each patient: dose at target depth, dose at surface (i.e., at 1 mm tissue depth), and irradiated surface (cm2).
Complications
Follow-up was performed according to the Dutch national guidelines for colorectal cancer [17]. Endpoints included all complications and readmissions within 30 days after surgery as well as all major post-operative complications 90 days after surgery. Analyses were performed separately for LARC and LRRC. Perioperative and post-operative complications were retrospectively classified using Clavien-Dindo classification [18, 19]. The highest Clavien-Dindo grade was noted for each patient up to 30 days after surgery. Major post-operative complications (Clavien-Dindo grade 3b-5) were additionally classified at 90 days post-operatively. From September 1, 2021, adverse events were routinely assessed by a radiation oncologist with common terminology criteria for adverse events, version 5.0 (CTCAE) at 3 and 12 months post-IOERT. These data were used to assess any long-term post-operative neuropathy. If not available, patient records were reviewed for evidence of peripheral neuropathy. No neuropathy was only classified if it was explicitly stated that no peripheral neuropathy was present.
Statistical analysis
Continuous data were expressed as a median (interquartile range – IQR) or mean (standard deviation – SD), as appropriate. Categorical data were presented as absolute numbers with percentages. Comparisons of continuous data were performed using independent t test, Wilcoxon sign rank test, and Mann-Whitney U test, as appropriate. Comparisons of categorical data were performed with chi-square test and Fisher-exact test, as appropriate. Two-sided p-values of < 0.05 were considered statistically significant. Statistical analyses were performed using IBM Statistical Package for the Social Sciences (SPSS) Statistics for Windows, version 29.0 (IBM Corp. Released 2022, Armonk, NY: IBM Corp.)
Results
Locally advanced rectal cancer
Between January 1, 2019 and July 31, 2023, 101 patients underwent surgery, of whom 54 (53%) were treated in the control group, and 47 (47%) in the (dose-escalated) intervention group. There were no significant differences in baseline characteristics, as summarized in Table 1. Surgeries performed were similar among treatment groups (p = 0.248) (Table 2). Additional treatment characteristics of LARRC patients with metastatic disease can be found in Supplementary Material. Overall, patients in the intervention group underwent fewer additional resections than patients in the control group (13% vs. 32%, p = 0.025), and significantly more pelvic sidewall resections were performed in the intervention group (40% vs. 19%, p = 0.015). Of the 54 patients in the control cohort, 34 (63%) received 10 Gy, 9 (17%) received 11.5 Gy, 7 received 12.5 Gy (13%), and 4 received 14.4 Gy (7%), both at surface and at depth. All patients in the intervention group received 10 Gy at depth, but obtained significantly higher IOERT dose at tissue surface: 15.0 Gy (n = 1; 2%), 15.5 Gy (n = 20; 43%), 16 Gy (n = 26; 55%), with a median of 16 Gy (IQR, 15.5-16) vs. 10 Gy (IQR, 10-12) in the historical cohort (p < 0.001). The irradiated surface was similar (p = 0.13).
Table 1
Baseline, tumor, and neoadjuvant treatment characteristics of patients with LARC and LRRC
Table 2
Surgical and intra-operative radiation therapy characteristics of patients with locally advanced rectal cancer (LARC) and locally recurrent rectal cancer (LRRC)
[i] LAR – low anterior resection, APR – abdominoperineal resection. Missing data were excluded from group comparisons; Due to rounding, not all percentages added up to 100%; Only men were included in group analysis of prostate and vesicle resections. No significant differences were reported in resections of the ureter, sacrum, lymph nodes, uterus, ovaries, or vagina
Table 3 shows comparisons of complications and readmissions for all patients. In LARC patients, the length of stay was shorter in the intervention group (7 days vs. 10 days, p = 0.010). There were significantly fewer 30-day post-operative complications in the intervention group (80% vs. 55%, p = 0.009), but the type of complications did not differ. Importantly, there was no increase in infectious gastrointestinal complications (p = 0.267) or wound complications (p = 0.748). Major post-operative complications were reported in 19% of patients, with no difference between treatment groups (20% vs. 17%, p = 0.761). There was no difference in complications at 90 days post-operatively (11% vs. 23%, p = 0.142), although data were missing in 24% of patients, mainly in the control group (30% vs. 17%).
Table 3
Group comparison of post-operative complications and readmission rates
Locally recurrent rectal cancer
Between January 1, 2019 and July 31, 2023, 99 patients with LRRC underwent surgery, of which 45 (45%) were treated in the intervention group. The baseline characteristics of LRRC are presented in Table 1. The characteristics of the primary tumor characteristics are shown in Supplementary Material. No differences in baseline characteristics were observed. However, patients in the intervention cohort received significantly less induction chemotherapy (78% vs. 29%, p < 0.001), but neoadjuvant CRT was similar (p = 0.392).
Full details of surgery and IOERT treatments are given in Table 2. Forty-nine percent of patients in the intervention group underwent a TE compared with 19% in the control group (p = 0.006). Significantly more bladder (53% vs. 17%, p < 0.001) and prostate resections (63% vs. 27%, p = 0.003) were performed in the intervention group, partly due to the higher number of TEs performed. Although the operations were more extensive, there was no difference in the duration of surgery (p = 0.674).
Thirty-seven (67%) patients in the control group received 10 Gy IOERT, 6 (11%) received 11.5 Gy, 7 received 12.5 Gy (13%), and 5 received 14.4 Gy (9%), both at the surface and at the specification depth. The dose at the depth was 10 Gy for all patients in the intervention group, but the dose at the surface was significantly higher, i.e., 15.5 Gy (n = 26; 58%) and 16 Gy (n = 19; 42%), with a median of 15.5 Gy vs. 10 Gy (p < 0.001). The irradiated surface was larger in the intervention group (40 cm2) than in the control group (28 cm2) (p = 0.009). The overview of the applicator diameter and bevel used is presented in Supplementary Material.
Post-operative complications were common but similar, occurring in 74% and 76% of patients, before and after dose adjustment, respectively (p = 0.866). Major post-operative complications occurred in 18 patients before (33%) and in 11 patients after (24%) dose adjustment (p = 0.624) at 30 days. There was no difference in the type of complications. Also, no difference was found in major complications at 90 days (p = 0.977), but data was missing in 32% and 22% patients from the control and intervention groups, respectively.
Peripheral neuropathy
Peripheral neuropathy was reported in 26 patients (15%) within 30 days, and in 30 patients (21%) within 90 days of surgery, as shown in Table 4 and Figure 3. An increase in reported neuropathy was observed at 12 months post-operatively in the intervention group (28%) compared with the control group (8%) (p = 0.019), but not at 30 or 90 days post-operatively. Data were missing in 15% at 30 days, in 30% at 90 days, and in 59% of patients at 12 months post-operatively. No CTCAE grade 4 or 5 neuropathy was observed. Operation reports were reviewed to investigate possible effects of surgery. In 12 patients (48%), neuropathy can be explained (in part) by the explicitly mentioned surgical resection of nerves or plexuses. Ten patients reported peripheral neuropathy at baseline that was due to chemotherapy or previous surgery. Peripheral neuropathy over time is shown in Supplementary Material.
Discussion
The current study suggests that increasing the IOERT surface dose in LARRC patients does not lead to an increase in post-operative complications. These results are in line with expectations expressed when the technique adaptation was introduced [16]. In Voogt et al. study, significantly more major complications occurred in LRRC patients treated with IOBT than with IOERT [14]. These complications included pre-sacral abscesses (26%), abdominal wound dehiscence with evisceration (8%), and intra-abdominal abscesses (6%). Several possible explanations were mentioned, such as a larger irradiated area with IOBT and a slower dose fall-off of IOBT, meaning that a larger volume of tissue received a higher dose. Operating times were also generally longer, as more time is required for applicator modulation and treatment planning. Another reason could be the higher surface dose of IOBT, with more heterogeneity within the surface dose and more hotspots. However, in this study, no increase in post-operative complications was observed despite an increase in the surface dose. This may indicate that the increased toxicity was caused by the other reasons mentioned.
In this study, there was a visible trend towards a larger irradiated area in the intervention group. However, the IOERT-irradiated volume was still smaller than that of IOBT. Previous studies have shown IOBT volumes on average 2-3 times larger than IOERT, with an upper limit exceeding 200 cm2 [14, 20-22]. Therefore, the relatively small irradiated volume in IOERT may explain why no increase in complications was observed. An alternative hypothesis could be that although the surface dose was increased, the dose fall-off was steeper because of the dose build-up within the PMMA bolus (Figure 1). Moreover, the IOERT dose in the historical cohort was scaled up to 14.4 Gy to increase the surface dose; however, owing to the physical properties, this also led to an increased dose beyond one centimeter depth. Therefore, with the PMMA bolus applied, theoretically, the dose at depth was less than that in the historical cohort.
Several factors complicated comparisons in this analysis. Due to the initiation of PelvEx II trial in LRRC, the proportion of patients receiving induction chemotherapy decreased, as induction chemotherapy was the standard of care for LRRC prior to PelvEx II study in the CHE [23]. This may have decreased the perioperative morbidity, concealing the possible increase in toxicity due to IOERT. Although data on toxicity due to pre-operative chemotherapy is lacking in LRRC, RAPIDO trial with LARC patients reported no increase in post-operative complications after 6 cycles of CAPOX [7]. Moreover, in the current study, the proportion of patients treated with induction chemotherapy in LARC showed an increasing trend (44% vs. 60%, p = 0.129), in part due to MEND-IT trial investigating the benefit of FOLFOXIRI induction chemotherapy in LARC [24]. Nevertheless, in patients with LARC, no increase in toxicity was observed. Therefore, the confounding effect of induction chemotherapy on perioperative and post-operative complications may be minimal. Prospective data generated from both the trials will provide more insight on induction chemotherapy-related complications [23, 25].
The differences in surgical techniques further complicate the comparison. In patients with LARC, the proportion of LAR vs. APR vs. TE was consistent. In LRRC, resections were significantly more extensive in the intervention cohort, due to an increase in TEs (19% vs. 49%). This increase may be related to a cohort study comparing LRRC management between the Karolinska Institute (KAR) and CHE, revealing more R0 resections at KAR (76% R0 vs. 61%), hypothesized to be due to more TEs (KAR 25% vs. CHE 16%, p = 0.02), with no difference in major complications (KAR 32% vs. CHE 30%, p = 0.742) [26]. On the one hand, more extensive resections could lead to an increase in complications and length of surgery. On the other hand, a TE is an en bloc resection, whereas operating more conservatively may result in additional partial resections, possibly leading to more tissue-healing complications. Nevertheless, no difference in complications was observed when stratifying for IOERT technique in either LARC or LRRC patients, despite varying operative management, supporting the idea that IOERT dose adaptation did not influence complications.
Peripheral neuropathy is an area of concern. The literature states that 3-23% of patients suffer from peripheral neuropathy due to extensive surgery and radiation within the pelvis [27, 28]. Clinically, there seems to be underreporting of peripheral neuropathy, and it should be expected that this is also true in the current study. Although the data were limited, there was an increase in peripheral neuropathy in the intervention group at 12 months. However, shortly prior to introducing the dose adaptation, a routine evaluation of peripheral neuropathy via CTCAE scoring at three months and one year post-operatively was performed. Therefore, it is possible that the increase in peripheral neuropathy is merely due to the improved reporting rather than due to the increased surface dose. Other factors influencing neuropathy are the surgery itself and chemotherapy [29]. As reported, neuropathy may also be explained by the performed surgery in several patients, as damage to the nerves or plexus within the pelvis was specifically mentioned in the surgical report. However, in reality, this percentage may be higher. When combining these factors, drawing conclusions, even on the extent of peripheral neuropathy as well as the difference in neuropathy between IOERT techniques, is challenging. Standardized CTCAE scoring of peripheral neuropathies will help improving our understanding of this potentially invalidating toxicity following IOERT. Neuropathy should remain an area of caution, as it can lead to severe decrease in quality of life [29-31].
One potential drawback of the new technique is the compromised view of target volume due to the PMMA bolus attached at the applicator exit. Adequate inspection of the at-risk volume and surrounding healthy tissue is necessary, with thorough multidisciplinary communication to deliver IOERT accurately and safely. Target volume reproducibility is also challenging, and may impede future treatment planning or understanding of IOERT-related complications. Intra-operative navigation may be able to provide a solution by facilitating pre-operative planning on MRI, intra-operative target volume definition, and post-operative dose reconstruction [32-35].
Ultimately, the goal is to improve LRFS. In Voogt et al. study, a significantly higher LRFS was reported after IOBT versus IOERT [14]. There are many differences between IOERT and IOBT that may have influenced these results, such as larger irradiated volumes or flexible applicator in IOBT, allowing for closer coverage of the at-risk area [16]. However, the fact remains that the at-risk area is generally at the tissue surface, at the resection margin. Therefore, it was hypothesized that the higher surface dose for IOBT could have contributed largely to these results. Several papers, including that of Appelt et al., indicate a dose-response relationship for tumor regression in rectal cancer [36]. A higher surface dose could translate to a more effective eradication of the remaining microscopic disease. A longer follow-up is needed to evaluate oncological outcomes.
This study has several limitations. Due to the recent introduction of the new technique, and the fact that patients are often referred to their own hospital after surgery, follow-up is often short, limiting conclusions. As follow-up data were mainly missing in the control group, there was a risk of bias and underreporting of complications in the control group; although there is no reason to suspect that the missing data would significantly influence the results. The introduction of the PelvEx II trial and the shift in surgical approach have both caused a significant difference in the characteristics of patients with LRRC. Nevertheless, it is unlikely that a clinically relevant, severe increase in toxicity due to IOERT dose adaptation would be missed because of the mentioned biases.
Although some conclusions can be drawn regarding safety of dose adaptation, no conclusions can be drawn regarding oncological effects. A longer follow-up period in a larger cohort is necessary. Additionally, a larger cohort will be needed to draw conclusions on more subtle complications, such as peripheral neuropathy that can be detrimental to quality of life, but are notoriously difficult to evaluate retrospectively. Standardized CTCAE scores during follow-up will facilitate future toxicity comparisons. Forthcoming work will also consist of analyses of oncological outcomes, especially of (in-field) LRFS, providing more information on the efficacy of dose adaption.
Conclusions
An increased surface dose of IOERT did not appear to increase post-operative complications. Further research is needed to evaluate the efficacy of dose adaptation of IOERT in improving the local oncological control rates. Routine evaluation of CTCAE scores during follow-up will help uncover possible long-term radiation-induced toxicities.